Traits
Trait: BCAAs and Insulin (PPM1K)
Dr Haran Sivapalan
/
June 29, 2020
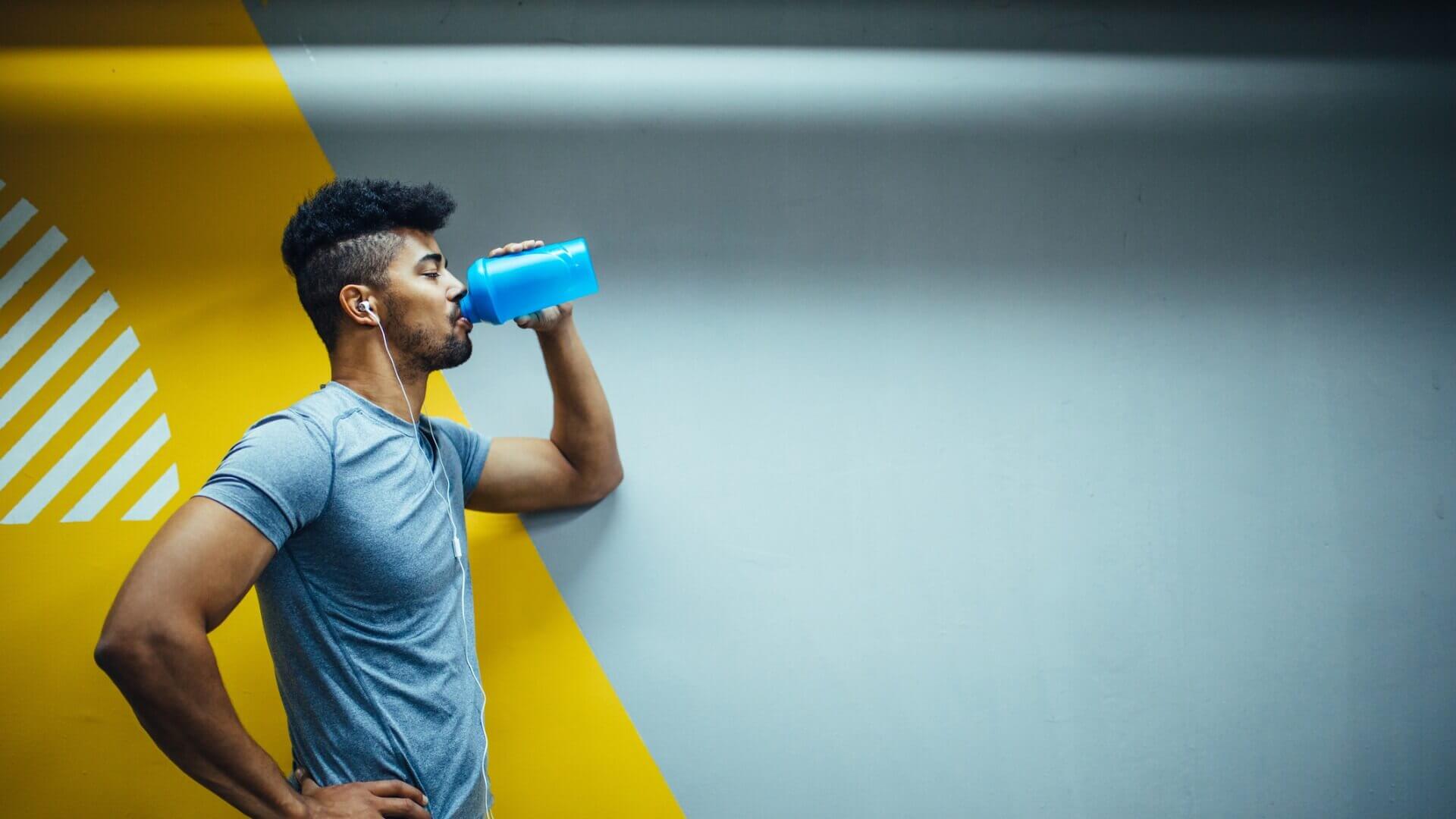
What are BCAAs?
BCAAs is an acronym for branched-chain amino acids. This is a group of three essential amino acids – leucine, isoleucine and valine.
To go over some basics, amino acids are the building blocks of proteins. The human body uses 21 amino acids, and many of these can be made by the body itself. Nine of these amino acids, called essential amino acids, cannot be made by the body and must be obtained from the diet. Leucine, isoleucine and valine (i.e. BCAAs) are all essential amino acids that we need to get from food and/or supplements.
BCAAs differ from other amino acids both in their structure and metabolism. Structurally, as their name suggests, BCAAs have chains that branch off to one side.
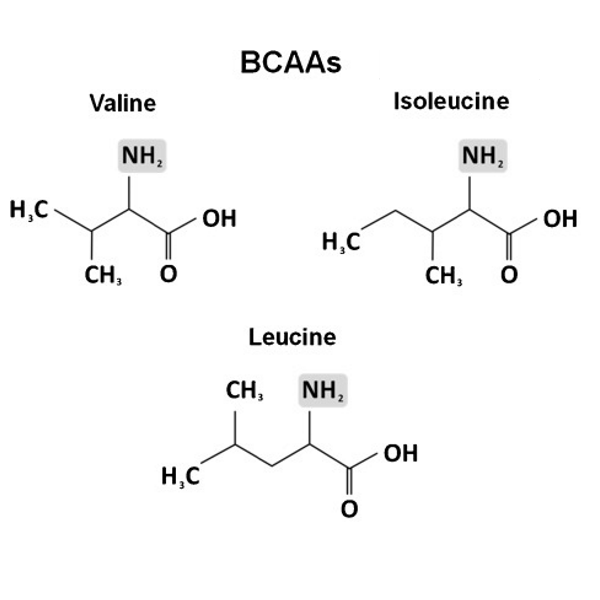
In terms of metabolism, BCAAs are unique in that they are predominantly broken down in muscle tissue, as opposed to the liver.
KEY POINTS
- BCAAs (Branched chain amino acids) are a group of three amino acids: leucine, isoleucine and valine.
What are the roles of BCAAs in the body?
If you’re interested in strength training and exercise nutrition, you may have come across BCAAs before in the context of supplements for building muscle.
In addition to stimulating muscle growth, BCAAs play several other key roles in the body, including regulating how tissues metabolise glucose and control blood sugar levels.
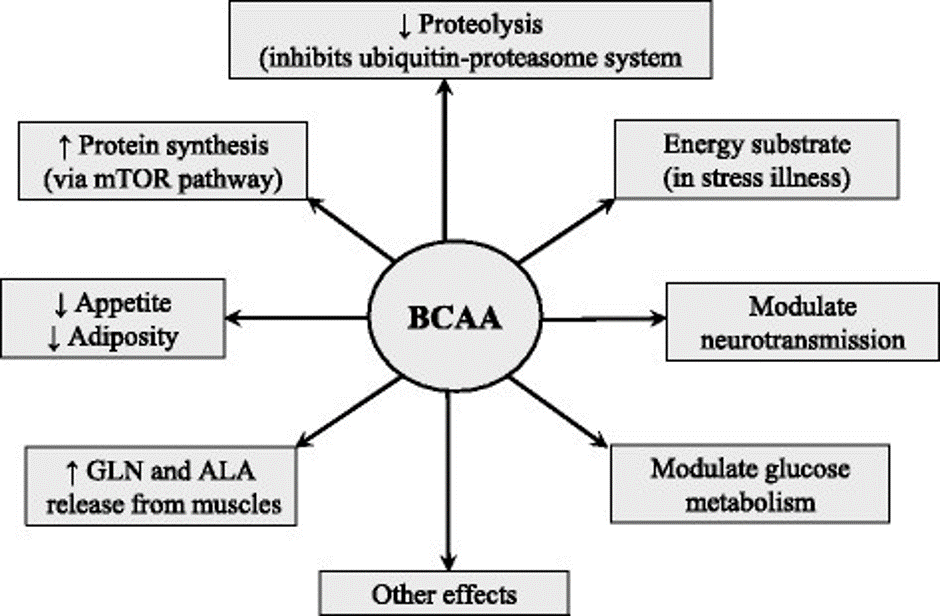
Protein Synthesis
BCAAs serve as building blocks for larger proteins, including those proteins that make up muscle fibers. Furthermore, BCAAs also directly stimulate the process of muscle protein synthesis, which can lead to muscle gains.
For example, leucine directly stimulates a cascade of chemical reactions called the mTOR signalling pathway, which leads to muscle protein synthesis. To find out more about this pathway and growth in muscle size and strength, please visit the Protein Synthesis and Hypertrophy (mTOR) article.
In addition to stimulating protein synthesis, BCAAs also inhibit the breakdown of protein (a process called proteolysis). In this respect, we say that BCAAs have an anabolic effect in the body – they promote the building up of molecules, cells and tissue.
Energy production
BCAAs can be used as an energy source by muscles, particularly when supplies of glycogen are low. Due to this effect, BCAAs become a useful energy source in long-duration, endurance exercise.
Similarly, BCAAs provide energy during times of starvation, trauma and illness.
Brain signalling
Neurotransmitters are nerve-signalling molecules which transmit messages from one nerve (or neuron) to another. BCAAs are transported into the brain and influence the production of various neurotransmitters, including dopamine, noradrenaline and serotonin.
On a similar note, BCAAs can reduce appetite by influencing the function of brain circuits that control food intake.
Control of glucose metabolism
BCAAs influence our metabolism of glucose and control of blood glucose (blood sugar) levels. On this note, BCAAs are shown to directly facilitate the entry of glucose into tissues, including liver and skeletal muscle.
Furthermore, BCAAs may stimulate the secretion of insulin. Insulin is the hormone that allows glucose to taken up from the bloodstream and used by tissues.
By both directly and indirectly (via insulin release) promoting the uptake of glucose, BCAAs can help to lower levels of glucose in the bloodstream.
Nevertheless, studies also show that high dietary intakes of BCAAs and high levels of BCAAs in the body can also impair the function of insulin. This can lead to elevated blood glucose levels, which may be damaging to health in the long term.
KEY POINTS
- BCAAs are often used as supplements to promote muscle growth and boost exercise performance.
- BCAAs stimulate protein synthesis in muscle.
- BCAAs are an important muscle energy source for long duration, endurance exercise.
- BCAAs play a role in the control of blood sugar levels.
- High levels of BCAAs may inhibit insulin function.
How do high BCAA levels affect glucose metabolism?
Several studies suggest that high levels of BCAAs can lead to a phenomenon called insulin resistance. This is where tissues do not respond properly to insulin and become insensitive to its effects. As a result of this, tissues struggle to take in glucose from the bloodstream, which can lead to elevated blood glucose levels.
Insulin resistance is linked to the development of obesity, Type II diabetes and metabolic syndrome (a cluster of high blood pressure, high blood glucose, excessive abdominal fat and unhealthy blood lipid levels).
On this note, people with these conditions have been shown to have higher tissue levels of BCAA compared to healthy individuals. Furthermore, evidence suggests that higher BCAA levels are associated a greater risk of developing Type II diabetes.
Why do high levels of BCAAs cause insulin resistance?
It is not clear how exactly high levels of BCAAs cause insulin resistance. We know that BCAAs cause stimulation of the mTOR signalling pathway. Excess stimulation of this pathway can impair insulin signalling, leading to poorer sensitivity to insulin.
Another potential explanation is that BCAAs interfere with the metabolism (beta-oxidation) of fatty acids in muscle tissue. This leads to build-up or intermediate metabolites, which impair insulin function and lead to insulin resistance.
KEY POINTS
- High levels of BCAAs may cause insulin resistance.
- Insulin resistance is a state where tissues are less sensitive to the effects of insulin, making it difficult for them to use glucose from the bloodstream.
- High levels of BCAAs are associated with the development of Type II diabetes and obesity.
What is PPM1K?
PPM1K is an enzyme with the full name: Protein Phosphatase, Mg2+/Mn2+ Dependent 1K.
It regulates the activity of another key enzyme – BCKD (branched chain α-keto acid dehydrogenase).
BCKD is involved in the breakdown and metabolism of BCAAs. Given this role, if the activity of the BCKD is reduced, less BCAAs are broken down leading to higher tissue levels of BCAAs.
KEY POINTS
- PPM1K is an enzyme that affects circulating levels of BCAAs.
How do PPM1K variants affect BCAA levels?
The PPM1K enzyme is encoded by the PPMK1 gene.
A SNP (Single Nucleotide Polymorphism) within the PPMK1 gene, given the code rs1440581, creates two different variants (or alleles) – the ‘T’ allele and the ‘C’ allele.
Studies suggest that the ‘C’ allele is associated with higher BCAA levels.
The potential mechanism by which the ‘C’ allele of the PPMK1 brings about higher BCAA levels is unclear. It is possible that inheriting this gene variant leads to reduced activity of the BCKD enzyme, and consequently lower breakdown of BCAAs.
KEY POINTS
- The ‘C’ allele (rs1440581) of the PPMK1 gene is associated with higher circulating levels of BCAAs.
How do PPM1K gene variants affect glucose metabolism?
The ‘C’ allele of the PPM1K gene is associated with a greater degree of insulin resistance and higher blood glucose levels.
Similarly, studies link the C allele to an increased risk of Type II diabetes.
It is thought that these effects on insulin resistance and diabetic risk result from higher levels of BCAAs linked to the ‘C’ allele.
Furthermore, evidence suggests that the effects of BCAAs on insulin resistance are worse when adopting a high fat diet.
KEY POINTS
- The ‘C’ allele (rs1440581) of the PPMK1 gene is associated with the development of insulin resistance.
- The ‘C’ allele is associated with a higher risk of Type II diabetes.
How do PPM1K gene variants affect weight loss and response to diet?
Variants of the PPM1K gene have been shown to affect your response to various diets. This phenomenon is known in biology as a gene x diet interaction.
More specifically, PPM1K gene variants influence weight loss and improvements in insulin sensitivity in response to diets with different fat content.
In a large clinical trial known as the POUNDS LOST trial, 734 overweight and obese individuals were split into different diet groups and followed over 2 years. The diet groups differed in their daily macronutrient ratios and, grossly speaking, could be classified as:
- A high-fat diet group – fat accounting for 40% of daily calorie intake.
- A low-fat diet group – fat accounting for 20% of daily calorie intake.
Researchers found that subjects responded differently to a high-fat diet, depending on what PPM1K variants they inherited.
As graph below shows, people carrying one or two copies of C allele (i.e. people with CC and CT genotypes respectively) experienced significantly less weight loss than those with the TT genotype in response to a high-fat diet.
Similarly, those with the ‘C’ allele showed poorer improvements in insulin sensitivity when adopting a high-fat diet.

By contrast, when fed a low-fat diet, people with the ‘C’ allele (CT and CC genotypes) responded much better, losing significantly more weight over the course of two years compared to those with the TT genotype. This is shown in the graph blow.
Furthermore, the ‘C’ allele was also associated with greater improvements in insulin sensitivity in the low-fat diet group.

Another clinical trial, known as the NUGENOB (Nutrient Gene Interactions in Human Obesity: implications for dietary guidelines) study, found that the ‘T’ allele was linked to greater improvements in insulin function in response to a high-fat (fat accounting for 40-45% of daily calories) diet only.
Both these findings suggest that people with the ‘C’ allele may lose more weight and experience greater improvements in insulin sensitivity when following a low fat diet.
Conversely, a high fat diet may benefit those with two copies of the ‘T’ allele, as they could potentially stand to lose more weight and show greater improvements in insulin sensitivity.
KEY POINTS
- Studies show the ‘C’ allele is associated with greater weight loss and improvements in insulin sensitivity in response to a low-fat diet.
- Individuals with two copies of the ‘T’ allele have been shown to have greater improvements in insulin sensitivity in response to a high-fat diet.