Traits
Trait: Metabolic Efficiency (UCP1)
Dr Haran Sivapalan
/
August 24, 2020
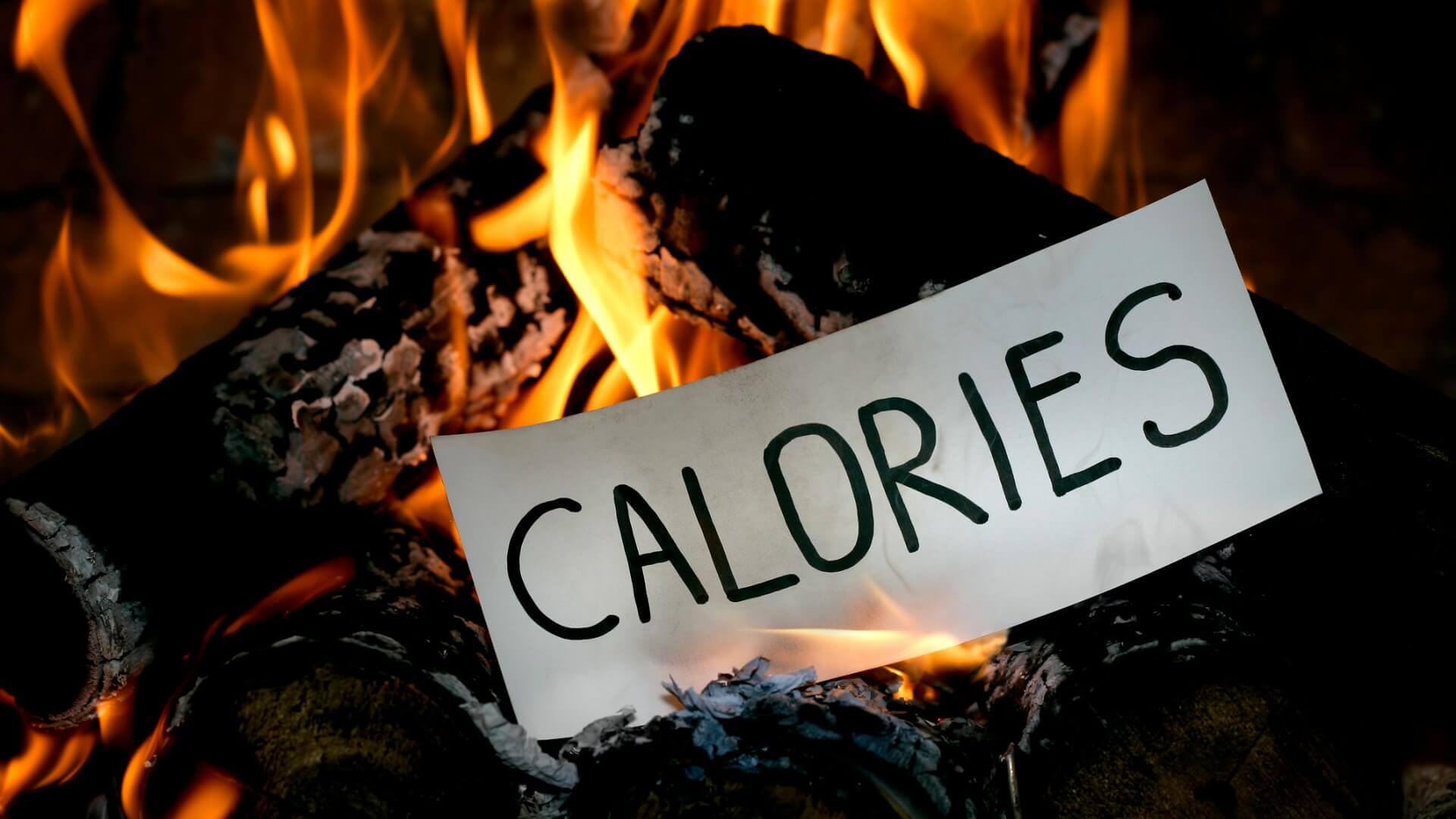
Metabolic efficiency
This trait looks at “metabolic efficiency” – a measure of how effectively your body converts chemical energy from food into chemical energy that can be used by cells for all the processes key to survival.
People described as having a “fast metabolism” actually have an “inefficient metabolism” – they ‘waste’ more chemical energy from food (or calories) as heat energy.
By contrast, people with a “slow metabolism” have a more “efficient metabolism” and convert a lower proportion of chemical energy into heat energy. The downside of an efficient metabolism is that excess energy from food is less likely to be wasted as heat energy, but more likely to be stored as fat, leading to weight gain.
Thermogenesis, the process by which our bodies generate heat energy, therefore plays an important role in our overall energy balance and risk of weight gain. It also plays a role in keeping us warm and the genes underlying non-shivering thermogenesis, such as UCP1, likely gave our ancestors a survival advantage in cold environments.
Why do we need to regulate body temperature?
Humans, like other warm-blooded mammals, need to maintain a fairly stable body temperature in order to survive.
If our core body temperature becomes too high, enzymes that carry out vital cellular reactions start to lose their structure and cease to work properly. Conversely, if our body temperature drops too low, then the rate of cell reactions becomes too slow in order to sustain life.
As a result of these constraints, we have evolved several different mechanisms to keep body temperature at an optimal level (around 37C).
For example, when we become too hot, our sweat glands release sweat. As sweat evaporates from the surface of our skin, it cools our body, helping to restore body temperature back to normal.
When we become too cold, hairs on our skin stand up (i.e. we get “goosebumps”), trapping a layer of air that insulates us and helps to keep us warm.
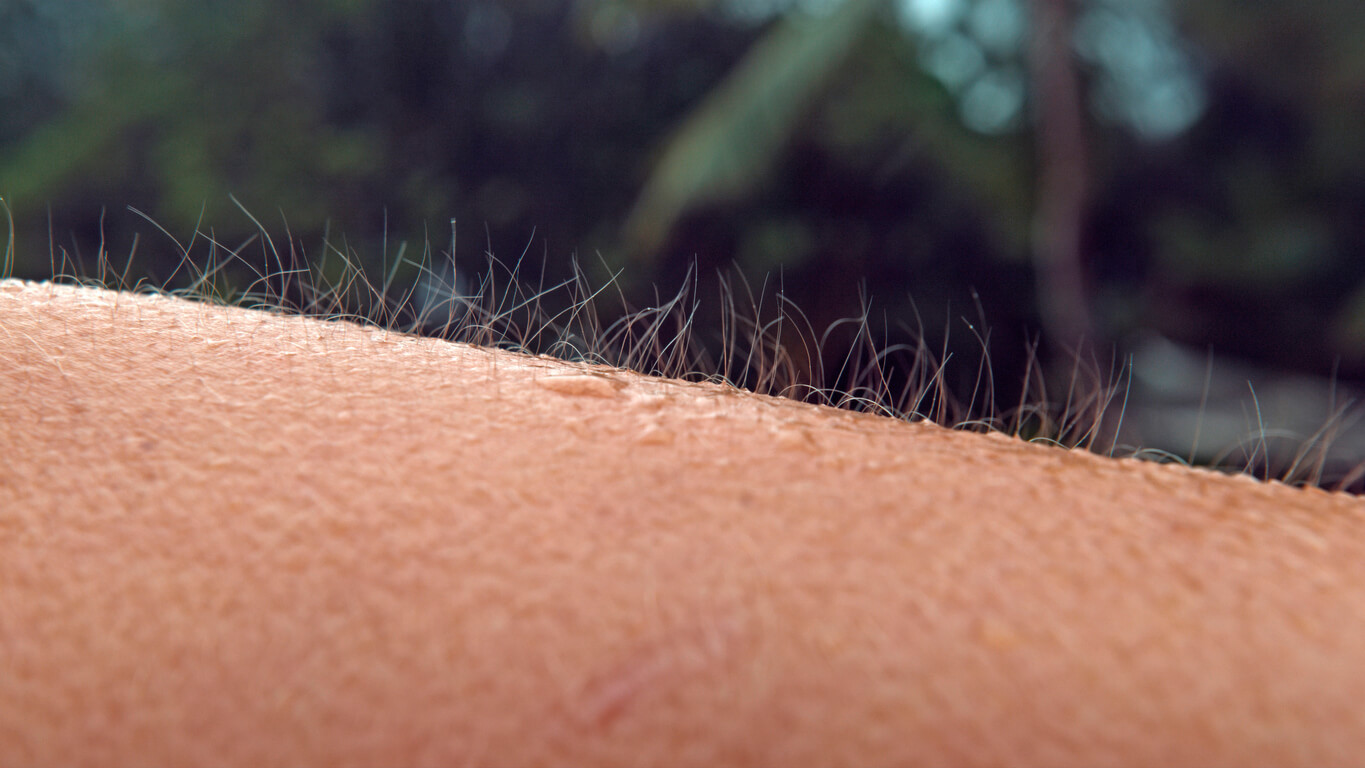
Both sweating and goosebumps are examples of thermoregulation – the process by which we maintain body temperature within a narrow range (typically between 36 and 38 degrees).
Thermoregulation itself is an example of a broader process in biology called homeostasis: - an organism’s maintenance of a constant internal environment.
KEY POINTS
- We strive maintain a core body temperature between 36 and 38 C.
- This is the optimal temperature for important chemical reactions and the effective function of enzymes.
- We have evolved various mechanisms to keep a stable body temperature.
How do we increase body temperature when it’s too cold?
When our body temperature drops, there are several ways by which we can warm up again. We’ve already mentioned “goosebumps”, whereby our hairs trap air from the environment to insulate us.
Our bodies can also generate their own heat energy. This is known as thermogenesis.
Thermogenesis is possible because metabolic reactions in our body (such as those in cell respiration, digestion and muscle contraction) are not 100% efficient - they generate heat energy as a by-product.
KEY POINTS
- Thermogenesis refers to the generation of heat from chemical reactions within the body.
Converting different forms of energy
If you can remember your high-school physics lessons, you may remember the first law of thermodynamics. According to this law: energy cannot be created or destroyed; it can merely be converted from one form into another.
Consider a light-bulb. This converts electrical energy into light energy. If, however, you’ve ever ill-advisedly touched a lightbulb, you’ll have noticed that it also gets considerably hot. This is because light-bulbs are inefficient: they do not convert all electrical energy into light energy. Instead, a significant proportion of electrical energy also gets converted or “lost” as heat energy.
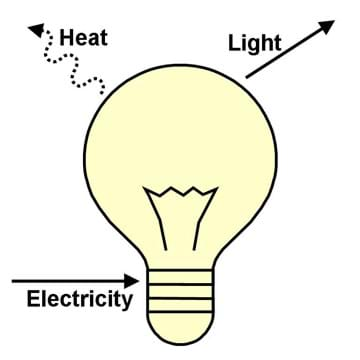
Our cells are similar. During respiration, they convert chemical energy from food into the common chemical energy currency, adenosine tri phosphate (ATP). ATP can then be used to fuel cell processes such as transport of molecules, growth and muscle contraction. A proportion of the chemical energy from food, however, gets converted into heat energy. This then increases our body temperature.
Muscle contraction also generates lots of heat energy. During muscle contraction, the chemical energy in ATP is converted into kinetic energy as our muscle fibers shorten. As part of this process, ATP is broken down by the enzyme myosin ATPase into ADP and phosphate. This chemical reaction produces a large amount of heat.
Incidentally, it’s for this reason that we get hot during exercise as lots of muscles contract. In fact, studies suggest that, compared to resting, our bodies produce 15-20 times more heat energy during exercise.
KEY POINTS
- Chemical reactions during muscle contraction generate heat energy.
- During cell respiration, cells convert chemical energy from food into ATP - our body's chemical energy currency.
- The process of cell respiration is not 100% efficient and also converts some chemical energy into heat energy.
Different types of thermogenesis
Working on the principle that metabolic reactions convert chemical energy into heat energy, there are two main types of thermogenesis:
- Shivering thermogenesis.
Shivering involves rapid, repeated contractions of our skeletal muscles. As explained above, the breakdown of ATP during muscle contraction generates heat energy.
- Non-shivering thermogenesis.
Non-shivering thermogenesis involves generating heat from metabolic reactions without muscle contraction.
In humans and other mammals, this largely involves diverting or “uncoupling” cell respiration to generate heat energy at the expense of generating ATP. Another way of looking it is to say that non-shivering thermogenesis makes cell respiration less efficient – a greater proportion of chemical energy is converted or ‘lost’ as heat energy.
As well as helping to keep us warm in the cold, non-shivering thermogenesis forms a major outlet for expending excess chemical energy consumed in food. Moreover, our bodies can alter the rate of non-shivering thermogenesis to regulate our overall energy balance. This is known as adaptive thermogenesis.
Thermogenesis and metabolic efficiency
Your Metabolic Efficiency and UCP1 trait focusses specifically on non-shivering thermogenesis.
Generally speaking, people with a lower metabolic efficiency have a higher rate of non-shivering thermogenesis and lose more energy from respiration as heat.
Conversely, people with a higher metabolic efficiency have a lower rate of non-shivering thermogenesis, and lose less energy as heat.
KEY POINTS
- Non-shivering thermogenesis involves generating heat energy without muscle contraction.
- During non-shivering thermogenesis, the process of cell respiration is made less efficient, so a greater proportion of chemical energy from food is converted into heat energy.
Which tissues are responsible for non-shivering thermogenesis?
Brown adipose tissue
Lots of mammals, including humans, carry a special kind of fat tissue called - brown adipose tissue (BAT). This fat tissue plays a key role in generating heat energy to keep us warm. The cells of BAT, called “brown adipocytes”, are uniquely designed to produce heat from cell respiration and produce a specialized protein called UCP1 in order to do this (we’ll learn about this later).
It used to be thought that brown adipose tissue was only present and used for thermogenesis in newborn infants. Mounting evidence, however, shows that brown adipose tissue is both present and metabolically active in adults. Nevertheless, the amount of brown adipose tissue we have declines with age.
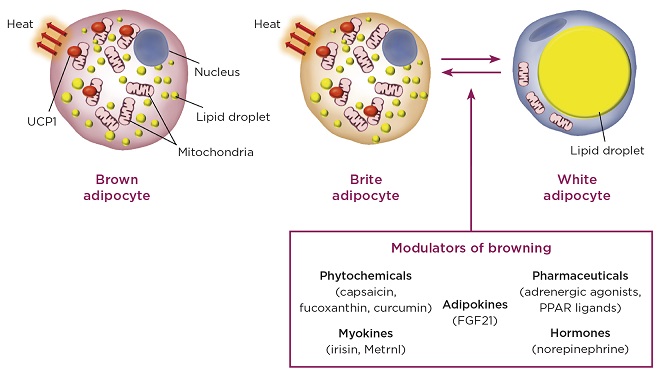
Beige-adipocytes
The other type of fat tissue that we carry is called white adipose tissue. This forms the majority of our fat tissue and acts as an energy store. White adipose tissue is composed mainly of cells called white adipocytes, which are formed of large lipid droplets and do not typically play a role in non-shivering thermogenesis.
Some white adipocytes, however, can become converted into “brown-like” adipocytes. In other words, they start producing the UCP1 protein and become capable of thermogenesis. These cells are known as “beige” or “brite” adipocytes.
The process of converting white adipocytes into beige adipocytes is known as “browning.” “Browning” is likely to be a beneficial adaptive response, and it occurs in response to sustained cold exposure and exercise.
KEY POINTS
- Non-shivering thermogenesis is carried out by brown adipose tissue.
- Brown adipose tissue is present and active in adult humans.
- White adipose tissue can undergo browning, where fat cells become capable of non-shivering thermogenesis.
How does non-shivering thermogenesis produce heat?
Brown adipose tissue is so-called because it appears brown, largely due to the presence of high amounts of mitochondria.
As you may recall from the Mitochondrial Biogenesis article, mitochondria are the “powerhouses of the cell”. Through a process of cell respiration, mitochondria convert the chemical energy from glucose, fatty acids and amino acids into the common chemical energy currency ATP.
The mitochondria of brown adipose tissue, however, produce a unique protein called uncoupling protein 1 or UCP1. This protein helps to “uncouple” cell respiration from the production of ATP, causing mitochondria to convert chemical energy into heat energy instead.
KEY POINTS
- Mitochondria in brown adipose tissue "uncouple" cell respiration from the production of ATP, generating heat energy instead.
Mitochondrial respiration
Before we go into detail about how mitochondria “uncouple” cell respiration to produce heat energy, it’s worth taking a look at how cell respiration ordinarily produces ATP.
Things get a bit complicated here, so we’ll try to keep everything as simple as possible.
Mitochondria are responsible for two stages of cell respiration in particular: the citric acid or Kreb’s cycle and the electron transport chain. (It is this final stage of respiration, the electron transport chain, that is used by mitochondria in brown adipose tissue to generate heat).
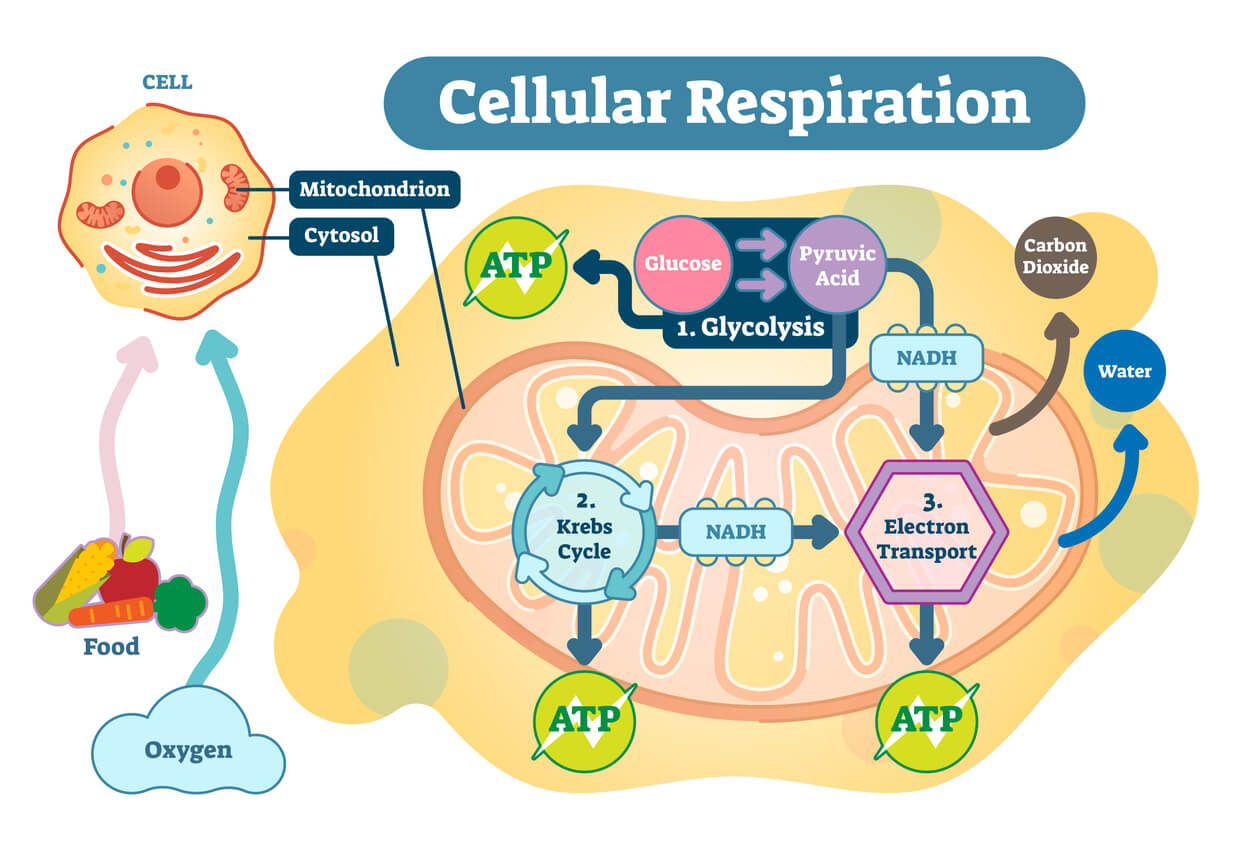
During the electron transport chain stage of respiration, mitochondria essentially convert electrical energy from the movement of charged particles into chemical energy in the form of ATP.
Mitochondria are specially designed for this function. As shown in the diagram below, mitochondria are composed of a matrix surrounded by an inner mitochondrial membrane. Outside of this membrane is a space called the intermembrane space. Finally, there is an outer mitochondrial membrane.
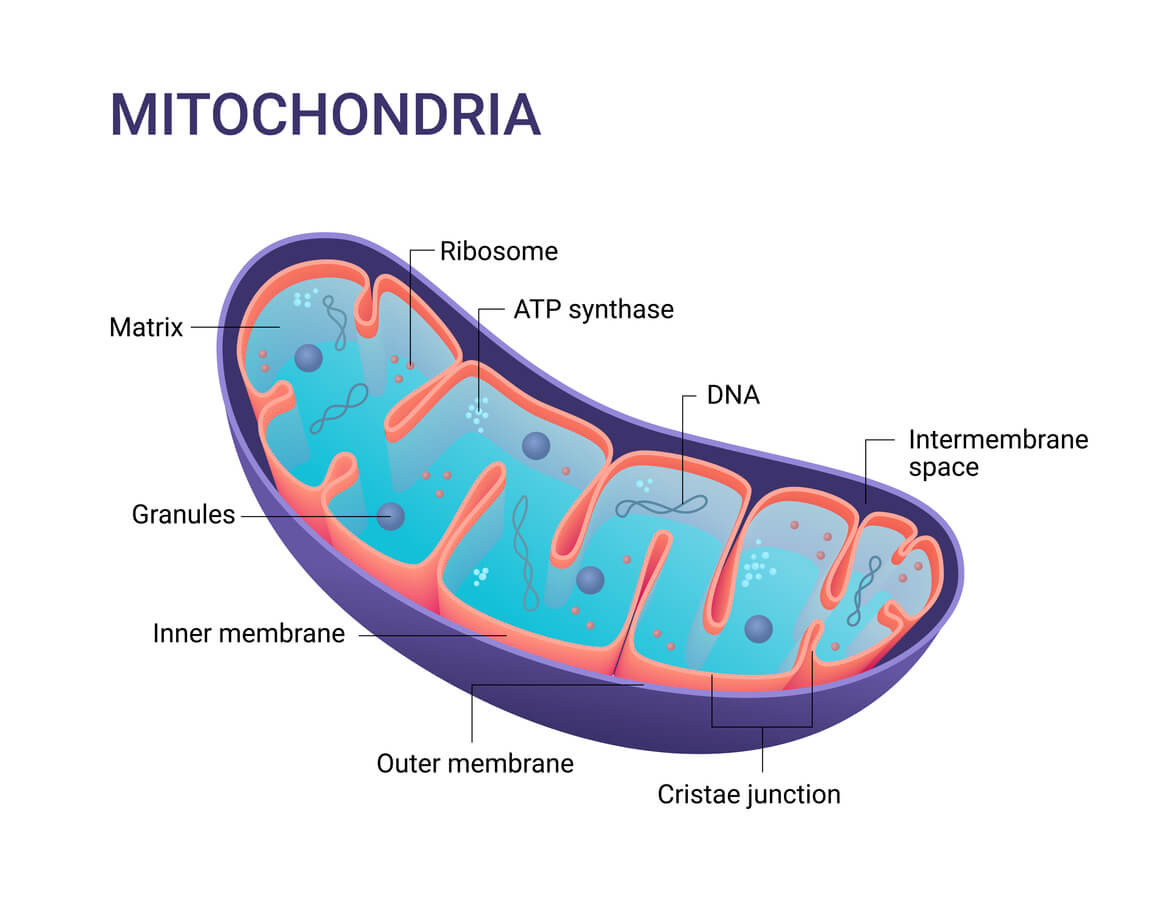
When hydrogen ions [H+] (also known as protons), which are particles carrying electrical charge, move across the inner mitochondrial membrane, ATP is generated.
More specifically, during the electron transport chain, H+ ions are pumped from the matrix across the inner mitochondrial membrane into the intermembrane space. This creates a voltage or potential difference – a bit like that in a battery.
The H+ ions are then allowed to move back (down a voltage gradient) into the matrix, but only through a specially designed enzyme called ATP synthase.
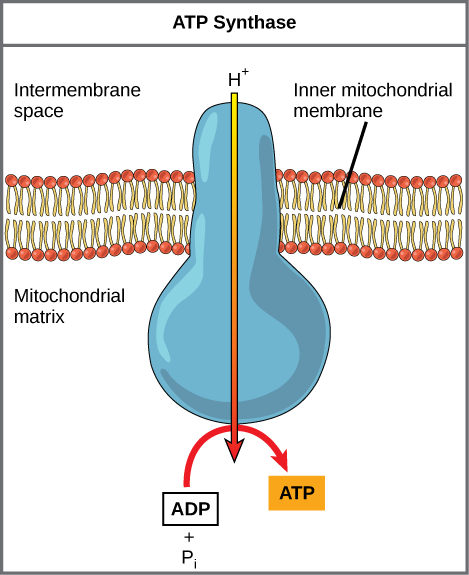
ATP synthase behaves a bit like a turbine in a dam. As hydrogen ions move back across the inner mitochondrial membrane, part of the ATP synthase enzyme spins like a rotor. This spinning movement then catalyzes the production of ATP – our cells’ energy currency.

In this respect, we say that the ATP synthase enzyme “couples” the movement of H+ ions (a form of electrical energy) to the production of ATP (a form of chemical energy).
KEY POINTS
- During cell respiration, mitochondria generate electrical energy - by pumping H+ ions from the matrix across the inner mitochondrial membrane.
- H+ ions are then allowed to flow back in through the ATP synthase enzyme.
- The ATP synthase enzyme converts electrical energy from the movement of H+ ions into chemical energy (in the form of ATP).
Mitochondrial uncoupling
As explained above, during cell respiration, H+ ions moving through the inner mitochondrial membrane are usually forced to go through the ATP synthase enzyme – thereby producing ATP.
In terms of energy conversion, this converts electrical energy into chemical energy.
The inner mitochondrial membrane, however, also has specialized channels called uncoupling proteins (UCPs). One UCP in particular, UCP1, is found in the mitochondrial membrane of brown adipocytes.
UCP1 allows H+ ions to move back into the matrix without going through the ATP synthase enzyme. It therefore “uncouples” the movement of H+ ions from the production of ATP.
This process is known as mitochondrial uncoupling or proton leak.
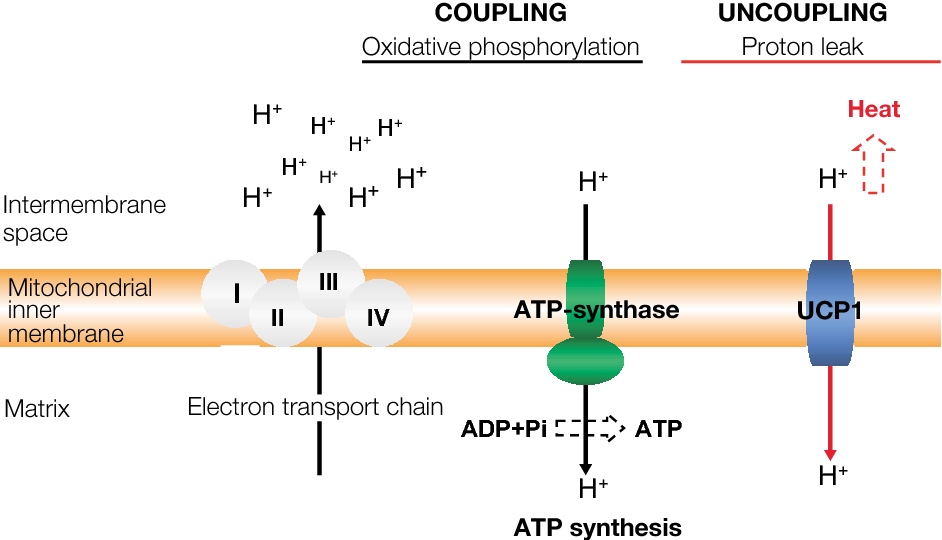
Again, in terms of energy conversion, this means less electrical energy gets converted into chemical energy.
According to the first law of thermodynamics, however: energy cannot be destroyed; only converted from one form into another. Consequently, instead of being converted into chemical energy, the electrical energy from the movement of H+ ions through uncoupling proteins gets converted into heat energy. This then warms the body up.
KEY POINTS
- UCP1 allows H+ ions to move across the inner mitochondrial membrane without going through the ATP synthase enzyme and producing ATP.
- UCP1 converts electrical energy from movement of H+ ions into heat energy.
- UCP1 reduces the conversion of electrical energy into chemical energy (ATP) - it uncouples cell respiration from ATP production.
What is UCP1?
UCP1 stands for uncoupling protein 1.
There are different types of uncoupling proteins (UCPs), including: UCP1, UCP2, UCP3, UCP4 and UCP5.
UCP1 is primarily involved in non-shivering thermogenesis and is unique to mitochondria in brown adipose tissue.
Furthermore, during “browning” of white adipose tissue, beige adipocytes start to produce the UCP1 protein and become capable of generating heat energy.
Activation of UCP1
UCP1 gets activated by fatty acids (which are one of the major building blocks of fat).
During non-shivering thermogenesis, brown adipose tissue breaks down fat into fatty acids. This process eventually feeds into the electron transport chain stage of respiration (as explained above), and causes H+ ions to be pumped across the inner mitochondrial membrane. As these ions then return through the activated UCP1 channels, they generate heat energy. Putting these steps together, we can think of brown adipose tissue as being able to literally “burn” fat to keep us warm.
Exposure to cold, exercise and stimulation of sympathetic nerves (which are responsible for the fight-or-flight response) may also stimulate fat burning in brown adipose tissue and activatie the UCP1 protein.
KEY POINTS
- UCP1 stands for uncoupling protein 1.
- UCP1 is unique to mitochondria in brown adipose tissue.
- Fatty acids generated from the breakdown of fat activate UCP1.
- Exposure to cold and exercise both activate and increase production of UCP1.
Uncoupling, metabolic efficiency and weight gain
“Calories in versus calories out”
You’ve probably heard that adage before, and while human metabolism is much more complicated than this simple line may suggest, it’s essentially true.
Whether we gain or lose weight depends on our overall energy balance: the difference between our energy intake from food (i.e. calories in) and our energy expenditure on processes we need to survive, grow and move (i.e. calories out).
Our energy balance is simply calculated with the following formula:
Energy balance = Energy intake – Energy expenditure
When our energy intake is higher than the amount of energy we expend (i.e. we have a positive energy balance), then we store the excess energy (predominantly as fat) and end up gaining weight.
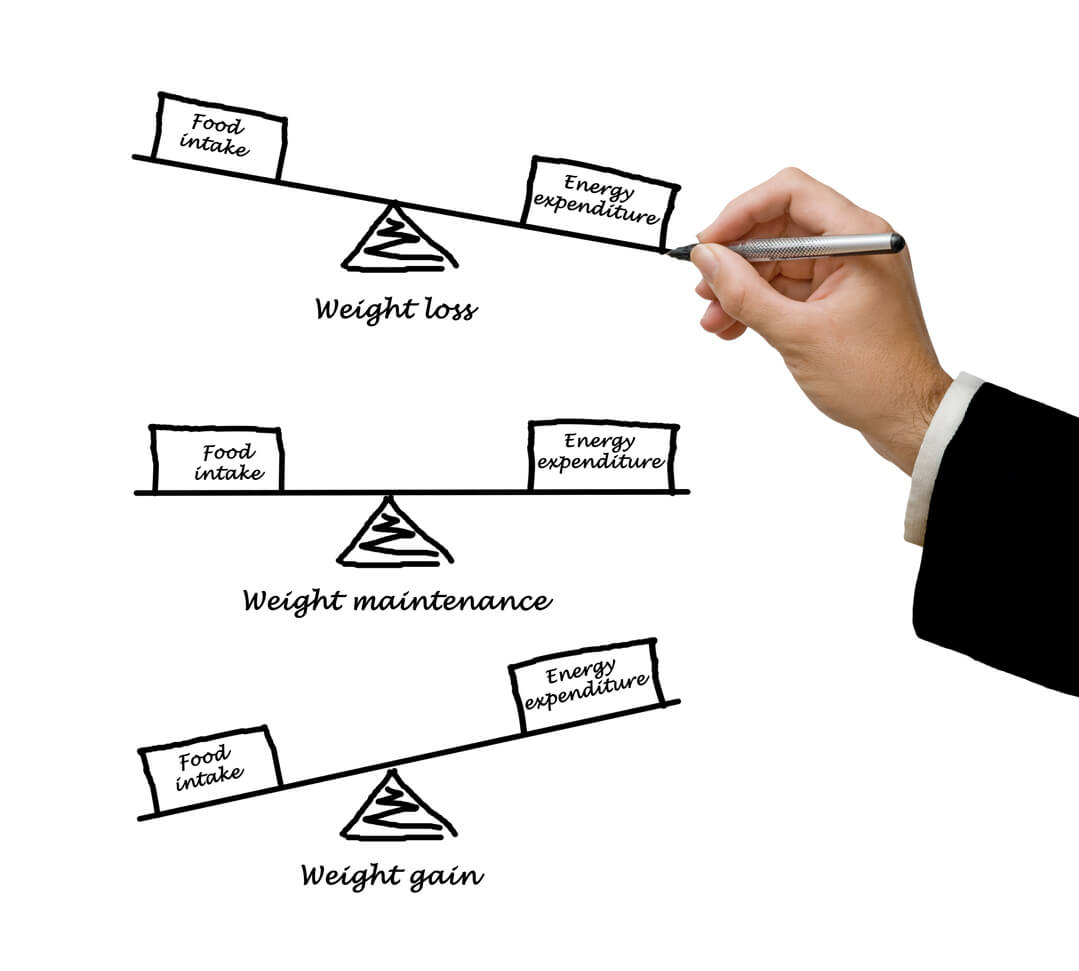
We know that our energy intake depends on what and how much food we eat. But, what exactly do we spend our energy on?
There are three broad sources of energy expenditure:
- Energy expended to digest and absorb food.
- Energy expended during physical activity.
- Energy expended on all the biological processes that keep us alive at rest (known as our basal metabolic rate).
Uncoupling / non-shivering thermogenesis makes up about 20-25% of our basal metabolic rate. It therefore constitutes a significant portion of both our basal metabolic rate and total energy expenditure.
Uncoupling and metabolic efficiency
If we have an “efficient metabolism”, however, we spend / “waste” less chemical energy on uncoupling to generate heat. This means our basal metabolic rate is lower and, all other things being equal, we will have lower total energy expenditure.
Going back to the energy balance equation, an “efficient metabolism” therefore makes easier for our energy intake to exceed energy expenditure. People with an efficient metabolism are therefore at greater risk of storing excess energy as fat and gaining weight.
By contrast, people with an “inefficient metabolism” spend more energy on uncoupling. They will therefore have a greater basal metabolic rate and, all other things being equal, a higher total energy expenditure. This puts them at lower risk of storing excess energy as fat and weight gain. The downside, however, is such individuals may less efficiently generate chemical energy for exercise and muscle building.
KEY POINTS
- Uncoupling / non-shivering thermogenesis to generate heat is a major source of energy expenditure.
- Uncoupling forms part of our basal metabolic rate - the amount of energy we burn at rest.
- People with an inefficient metabolism "waste" more energy on uncoupling, burn more calories at rest, which may lead to higher total energy expenditure.
- People with an efficient metabolism "waste" less energy on uncoupling, burn fewer calories at rest, which may lead to lower total energy expenditure.
- An efficient metabolism increases the risk of weight gain if energy (food) intake exceeds energy expenditure.
Genetics
Your latest trait analyzes variants of your UCP1 gene, which encodes the UCP1 uncoupling protein.
Variants of this gene affect the expression of the UCP1 protein in brown adipose tissue and therefore influence your rate of mitochondrial uncoupling / non-shivering thermogenesis. In turn, this affects your metabolic efficiency, which itself can alter your risk of weight gain.
Studies suggest that gene variants associated with lower expression and activity of UCP1 protein lead to less uncoupling and therefore more efficient metabolism. This increases the risk of overweight and obesity.
Regardless of your genes, there are several lifestyle changes you can make to alter the expression of UCP1 protein. Be sure to check out your personalized actions in the Metabolic Efficiency and UCP1 trait.
KEY POINTS
- Variants of your UCP1 gene affect your rate of non-shivering thermogenesis, metabolic efficiency and risk of weight gain.