Traits
Your Renin-Angiotensin-Aldosterone System and why it's important
Dr Haran Sivapalan
/
March 11, 2019
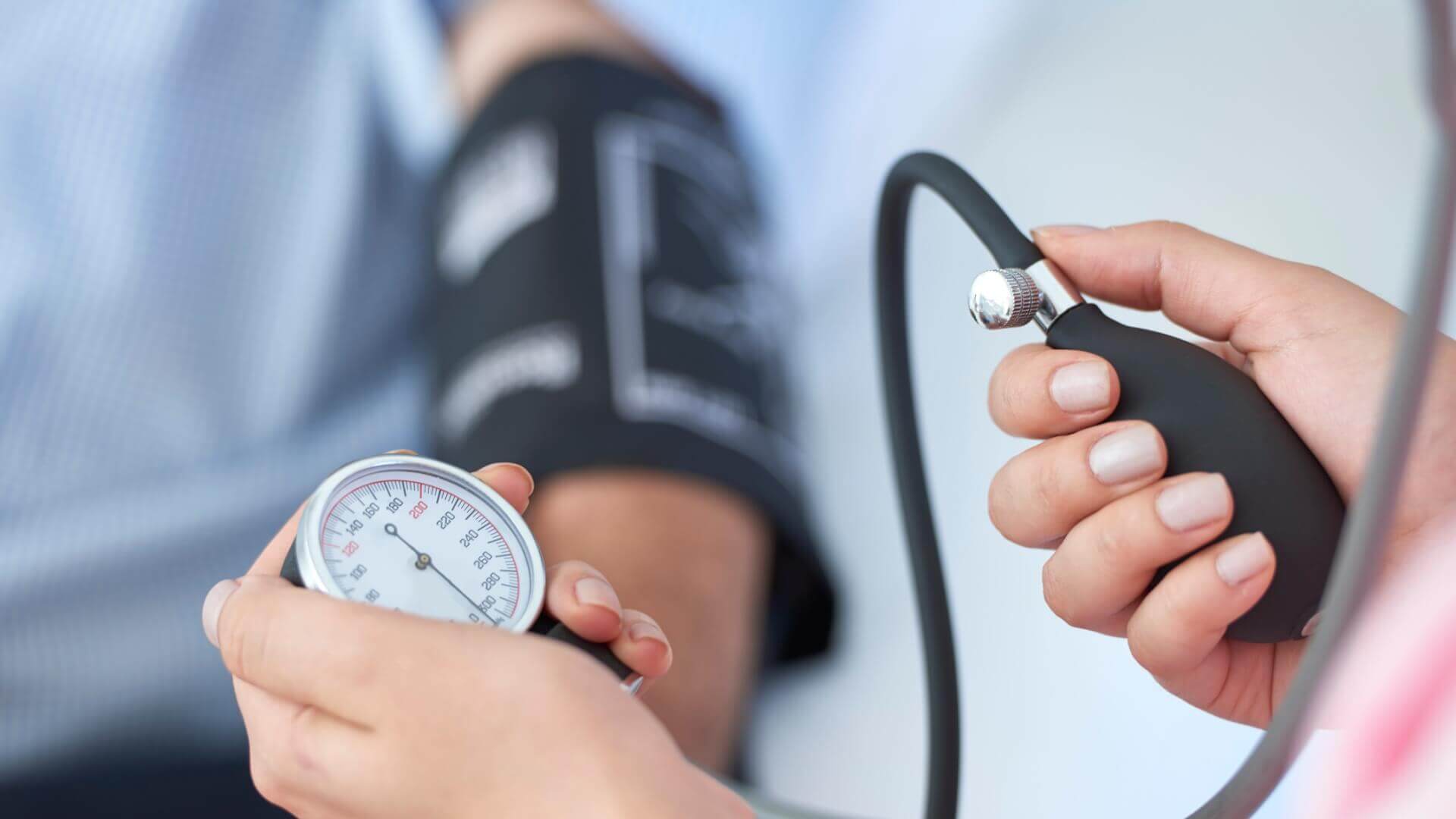
A word about homeostasis
Consider a thermostat in your house or office. This contraption is designed to keep rooms at a constant temperature. If the temperature suddenly drops (say during a cold winter night), the thermostat detects this and switches on radiators and heaters to warm the rooms and restore normal room temperature. If the temperature were to rise too high (say during a hot summer day), the thermostat will again detect this, but now will switch off the heaters and perhaps switch-on air conditioning to cool the rooms.
In the same way a thermostat acts to keep your rooms at a constant temperature, every biological organism, from a single-cell bacterium to a complex human body, strives to maintain a constant internal environment. We call this process “homeostasis.”
Even as you read this, your body is conducting various biochemical and physical processes to maintain temperature, water content, acidity/alkalinity (pH), minerals and blood sugar concentrations at a fixed level (or within a narrow range of levels). Collectively, we call these biochemical and physical processes homeostatic feedback mechanisms.
Two other crucial (and interrelated) parameters that we regulate through homeostasis are: blood pressure and blood volume. To control these two parameters, we’ve evolved a complex hormonal system involving communication between our brain, lungs, liver and kidneys. This hormonal system is known as the renin-angiotensin-aldosterone system (RAAS).
Key points
- Homeostasis is the regulation of a constant internal environment.
- We use homeostatic processes to regulate blood pressure and blood volume.
- The Renin-Angiotensin-Aldosterone System (RAAS) is a hormonal system (involving the brain, lungs, kidneys and liver) that helps us to regulate blood pressure and volume.
What is blood pressure and what is blood volume?
Before we explore the RAAS, it’s important understand why we need to homeostatically control blood pressure and volume in the first place. To do that, we first need to clarify the terms blood pressure and blood volume.
Blood pressure
Blood pressure simply refers to the pressure of the blood circulating in your blood vessels (arteries and veins). This pressure is created by the pumping action of your heart.
If you’ve ever had your blood pressure checked by a nurse or at the gym, you’ll have noticed that you received two figures e.g. 120/80 mm Hg or 110/75 mm Hg.
The first figure (i.e. 120 or 110 in the previous example) is known as your systolic blood pressure. This figure refers to the pressure in your vessels when your heart is contracting and pumping blood to your tissues and organs.
The second figure (i.e. 80 or 75) is known as your diastolic blood pressure. This is the pressure in your vessels when your heart is relaxing and refilling with blood. As you’ll notice, this pressure is lower than your systolic pressure because your heart isn’t contracting forcefully.
In order to simplify things into one reading, when we talk about blood pressure, we are referring to the average (mean) pressure in the blood vessels as your heart both contracts and relaxes. We call this mean blood pressure.
There is also a large difference between the pressure in your arteries and veins. Arteries are blood vessels that (with a couple of exceptions) carry oxygenated, nutrient-rich blood away from the heart to be used by tissues and organs. The pressure of blood in arteries (or ‘arterial blood pressure’) is therefore higher as it has been freshly pumped by the heart.
By contrast, the pressure in veins, which are vessels that return deoxygenated blood back to the heart, is quite low. Interestingly, due to the low pressure of venous blood, our veins have special valves to help return blood back to the heart. We also make use of muscle contraction (e.g. of the calf muscles) to help ‘pump’ blood back to the heart against the force of gravity.
Anyhow, to avoid complicating things, when we refer to blood pressure, we are typically just referring to the pressure in the arteries (arterial blood pressure).
As mentioned before, we take an average (mean) to account for both heart contraction and relaxation. Together then, whenever we mention blood pressure, we are specifically referring to mean arterial blood pressure.
Blood volume
Blood volume is the total volume of blood circulating in your heart, arteries, veins and capillaries at any one time. In the average human, this volume is approximately 5 litres.
The majority (about 60%) of your blood volume is made up of fluid called plasma. Plasma is largely made up of water, electrolytes (e.g. sodium and potassium), proteins and hormones. Owing to the large contribution of water and electrolytes to plasma, your blood volume is an indirect measure of your hydration status. If your blood volume is low, this may indicate a loss or inadequate intake of water and electrolytes. As we’ll find out later, your body has a host of homeostatic mechanisms to correct this.
Aside from plasma, the remaining 40% of your blood volume is made up of various blood cells, including red blood cells (which transport oxygen around your body), white blood cells (which help fight infection) and platelets (which help form blood clots to prevent bleeding).
How are blood pressure and blood volume related?
As mentioned in a previous blog, your circulatory system is essentially a pump (your heart) with a closed system of tubes (your blood vessels), in which fluid (blood) circulates. If you fill the system with more fluid, the pressure inside the tubes ought to increase. Consider inflating a bicycle tyre with air. As you pump more air into the tyre, the pressure inside the tyre increases.
In the same way, the higher blood volume circulating in your vessels, the higher your blood pressure.
Key points
- Blood pressure is the pressure at which blood is pumped through your blood vessels.
- We are mainly concerned with the average blood pressure in arteries, which supply organs and tissues with oxygenated, nutrient-rich blood.
- Blood volume is the total volume of blood circulating in your heart, arteries, veins and capillaries at any one time.
- Blood volume is approximately 5 liters in the average adult.
- Blood volume is related to your body’s water content and hydration status.
- Generally speaking: the higher your blood volume, the higher your blood pressure.
Why do we need to regulate blood pressure and blood volume?
All of our tissues and organs require oxygen, water and nutrients to survive. These substances are transported in the bloodstream via arteries. For tissues and organs to survive, then, they must receive adequate blood flow.
In the physical world, fluids generally flow down a pressure gradient – that is, from a region of high pressure to a region of low pressure. For example, when it comes to the weather, wind results from air moving from areas of high pressure to areas of low pressure. Or reconsider the bicycle tyre example. If you were to sustain a puncture, air will suddenly flow down a pressure gradient: from the high pressure inside the tyre to the lower pressure of the atmosphere outside.
Similarly, for blood to flow into organs and tissues, there must be a pressure gradient between the blood in arteries (arterial blood pressure) and blood in capillaries in an organ.
In other words, the pressure of blood in arteries (or, simply, blood pressure) must be high enough for blood to flow down a pressure gradient into organs, supplying them with oxygen and nutrients.
This is the fundamental reason we need to homeostatically regulate blood pressure – to ensure tissues and organs are adequately perfused with blood.
If blood pressure falls too low, then the pressure gradient will drop, causing reduced blood flow into tissues and organs.
Conversely, if blood pressure gets too high, then the heart must work harder to pump blood around the body. This places additional strain on the heart. If you’ve ever pumped up a bicycle tyre, you’ll notice that it takes more effort during the later stages as the pressure inside the tyre is higher.
An excessively high blood pressure also increases the risk of vessels bursting. Again, returning to the bicycle tyre example, if you overinflate it with air, it may burst. Burst blood vessels are not desirable – they can lead to blood loss, thereby impairing blood flow to target tissues and damaging to surrounding tissue.
And what about blood volume? As blood volume is intimately related with blood pressure, the same principles apply. In other words: low blood volume (which is associated with low blood pressure) will compromise blood flow to organs. At the opposite end, an overly high blood volume will put extra strain on the heart.
Additionally, recall that blood volume is an indirect measure of our hydration status. If blood volume is too low, it is a sign that your body requires more fluid and electrolytes to perform vital functions. If blood volume become too high, we need to get rid of fluid.
Key points
- Blood flows to organs and tissues through arteries down a pressure gradient.
- Blood pressure and blood volume need to be controlled to ensure organs and tissues are adequately perfused with blood.
- Low blood pressure and low blood volume impair blood flow to organs and tissues.
- High blood pressure and blood volume place strain on the heart.
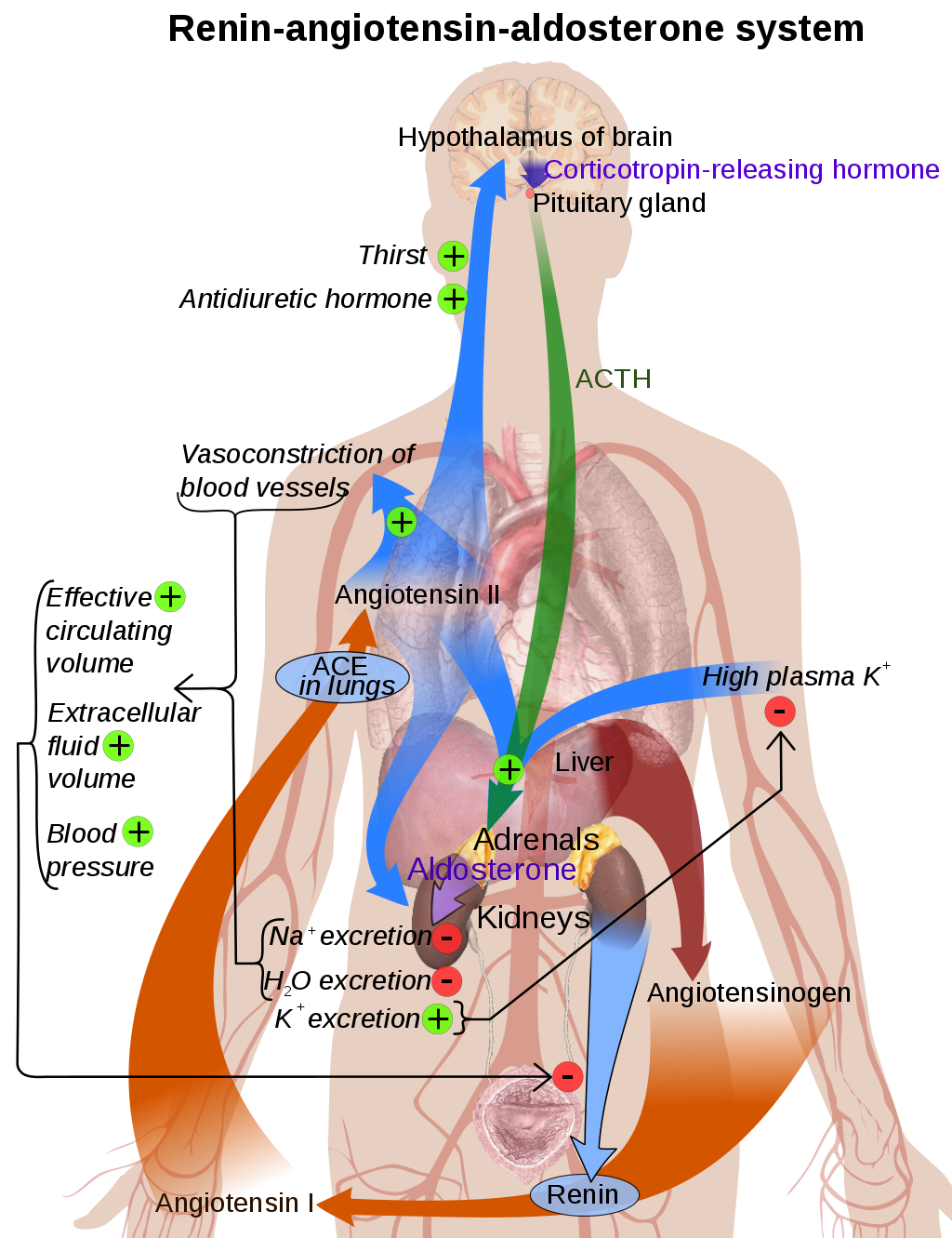
What happens when blood pressure / blood volume drops?
It’s an unpleasant scenario, but imagine you’ve just been mauled by a tiger and lost a lot of blood. Owing to the blood loss, your blood volume will decrease. As a lower volume of blood is flowing through your circulatory system, your blood pressure will also drop.
As we established earlier, a drop in blood pressure and volume will reduce blood flow to vital organs, such as your brain, gut and kidneys. In order to survive, your body will need to quickly restore blood pressure back towards normal. Before it can do this, however, it must detect the drop in blood pressure/volume in the first place.
- Sensing changes in blood volume and blood pressure
Your body has evolved various mechanisms to detect changes in blood pressure and blood volume.
Baroreceptors
Found in the walls of large arteries and veins, as well in the arteries supplying the kidney, are special blood pressure receptors called baroreceptors. Baroreceptors essentially work by detecting the degree to which blood vessel walls stretch – higher blood pressure leads to greater stretching of arterial walls. As your blood pressure drops due to blood loss, your baroreceptors will sense the reduced stretch of arterial walls.
Kidneys (Juxtaglomerular apparatus)
Your kidneys are also well-designed to monitor changes in blood pressure and volume. Now, as you’ve probably heard before, the basic function of your kidneys is to ‘filter your blood’ and create urine to be excreted.
When there is a fall in blood pressure, blood flow to your kidneys decreases. This causes less blood to be filtered and less urine to be created. Fortunately, your kidneys have a special structure called the juxtaglomerular apparatus, which can sense this reduction in blood flow and filtration rate. It does this in two principle ways.
Firstly, like your blood vessels, your juxtaglomerular apparatus also contains baroreceptors which sense reduced blood pressure in the arteries supplying the kidneys.
Secondly, when blood flow to your kidneys decreases and/or there is a drop in blood volume, less salt (sodium chloride) is filtered into the urine. Your juxtaglomerular apparatus has a dedicated collection of cells, called the macula densa, which can detect reduced sodium concentration in the urine.
- Renin release
Your kidneys do not just passively monitor changes in blood pressure and volume, they can respond to these changes too. Again, this is all part of a homeostatic feedback mechanism to maintain your blood pressure and volume within normal limits.
When baroceptors first sense a drop in blood pressure and the kidneys (specifically the macula densa) senses a decrease in sodium flow, they kick off a chain of processes to rectify the situation.
In the first stage of this chain, your baroreceptors and macula densa stimulate cells in your kidney to produce an enzyme called renin.
When renin is released into the bloodstream, it acts on another molecule, angiotensinogen. Angiotensinogen is an inactive protein circulating in the blood that is initially produced by your liver. Renin released from the kidneys acts on angiotensinogen, converting it into another protein – angiotensin I.
- Formation of angiotensin II
Angiotensin I itself doesn’t do much in the body. Instead, it is rapidly converted by an enzyme, Angiotensin Converting Enzyme (ACE), into a more biologically active molecule – Angiotensin II. This conversion process takes place in your lungs and kidneys, where the linings of blood vessels produce the ACE enzyme.
- Effects of angiotensin II
Angiotensin II is a potent, biologically active molecule designed to trigger mechanisms that restore and increase your blood pressure.
In this respect, it has four main effects in the body:
Vasoconstriction
As discussed in the previous blog, one key way to increase blood pressure is to increase the resistance to blood flowing in blood vessels. Angiotensin II is a potent vasoconstrictor – it causes arteries to become narrower. By doing so, angiotensin II raises blood pressure.
It’s important to note that while vasoconstriction is useful in acute, short-term acute situations (e.g. raising blood pressure in response to sudden blood loss), inappropriate degrees of vasoconstriction can impair blood flow to various tissues, including exercising muscles.
Sodium reabsorption
Sodium is an important electrolyte in the body and is sometimes referred to as ‘salt’. By controlling the movement of sodium in and out of tissues, your body can actually control the movement of water. In turn, by altering the movement of water, your body can adjust blood volume.
If you can remember your high school biology lessons, you’ll recall that water tends to move from a high solute (salt) concentration to a low solute concentration. We call this process osmosis.
Using this principle, angiotensin II causes cells in the kidney to reabsorb more sodium from the urine into the bloodstream. Through the process of osmosis, water follows the sodium into the blood. This has the effect of increasing the blood volume and elevating blood pressure.
Aldosterone release
Angiotensin II also acts on glands just above your kidneys, called the adrenal glands. These glands produce various different hormones, including adrenaline (the ‘fight or flight hormone’) and cortisol (the ‘stress hormone’). Under the influence of Angiotensin II, your adrenal glands produce another hormone called aldosterone.
Aldosterone works in a similar fashion to angiotensin II: it stimulates sodium reabsorption by the kidneys (see ii above). Specifically, it causes the kidney to produce specialised sodium channels to enhance the reabsorption of sodium into the bloodstream. It also stimulates the production of sodium/potassium pumps, which actively pump sodium back into the bloodstream in exchange for potassium (which is excreted into the urine).
Increased thirst and water reabsorption
Angiotensin II also acts on the brain, where it activates thirst centres. Consequently, you feel thirsty and are more likely to drink fluids, thereby increasing your blood volume. Similarly, Angiotensin II also works on your pituitary gland, causing it to release another hormone called ADH (Anti-Diuretic Hormone) [sometimes called ‘vasopressin’]. This hormone acts on the kidneys, increasing water reabsorption into the blood, and therefore producing more concentrated urine.
It’s worth noting that the above effects of Angiotensin II are largely brought about by angiotensin II binding to one particular class of receptor, the Angiotensin II Type 1 receptor or (AT1 receptor).
Key Points
- Your body has specialised pressure receptors (baroreceptors) and structures in the kidneys (juxtaglomerular apparatus) to detect a fall in blood pressure/ volume
- A fall in blood pressure/volume triggers a chain of events in the renin-angiotensin-aldosterone system (RAAS) to correct and restore blood pressure/volume.
- Angiotensin II is produced at the end of this chain of events.
- Angiotensin II causes: vasoconstriction, increased sodium reabsorption, increased thirst and production of another hormone, aldosterone.
- Aldosterone stimulates sodium reabsorption into the blood from the kidneys.
- Water tends to follow sodium, so reabsorption of sodium also increases the reabsorption of water.
- This increases blood volume and, therefore, blood pressure.
Genetics and individual differences in the RAAS
Genes play a role at all stages in the RAAS. Due to variation in these different genes, we all will have little differences in the way we regulate blood pressure and volume. Furthermore, due to such genetic variation, we may have to eat, exercise and live in different ways best suited to our particular genetic makeup.
Below are various stages where genes may exert differences in the RAAS system.
- Sensing changes in blood pressure/volume
In the same way some of us are more sensitive to different tastes, sights and smells, our baroreceptors (which sense pressure changes) have different sensitivities. This means that some of may respond more or less intensely to fluctuations in blood pressure and volume.
- Renin release
Your REN gene may affect the function and activity of renin. Interestingly, there may also be racial differences in renin activity, with African-Americans widely shown to have reduced renin activity.
Renin acts on angiotensinogen, which is encoded by your AGT gene. Variations of this gene, which you’ll find in your FitnessGenes DNA results, affect circulating levels of angiotensinogen. In turn, this may affect how you respond to changes in blood pressure and volume. Different versions of the AGT gene are also linked to differences in strength and muscle growth.
- Formation of Angiotensin II
Different versions of your ACE gene, which encodes Angiotensin Converting Enzyme, may also impact the activity in the RAAS system. Interestingly, the I variant (or allele) of the ACE gene is associated with lower activity of Angiotensin Converting Enzyme and therefore lower levels of Angiotensin II. This variant has also been linked to better endurance ability.
- Effects of Angiotensin II
As mentioned earlier, most of the short-term effects of Angiotensin II on blood pressure and volume are mediated by the AT1 receptor. The AT1 gene encodes this receptor and variations in this gene may affect the quantity of receptors produced by your body. In turn, this could influence your body’s response to of Angiotensin II.
There are also different genes involved with the production and function of aldosterone. One of these is the CYP11B2 gene, which encodes an enzyme used to make aldosterone. Variations in this gene affect the amount of aldosterone produced and have been linked to differences in blood pressure.